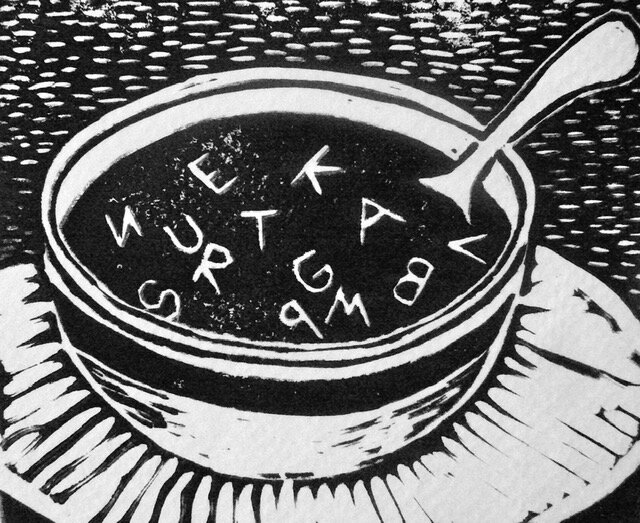
“The essential message of life has been copied and recopied for more than three billion years, but where did that message come from?”
Neil deGrasse Tyson, Cosmos: A Spacetime Odyssey.
Research and discussions about origins, whether about the universe, life, or our own ancestry, have a sense of convergence to them. They help narrow down the infinite number of potential explanations to a select few or maybe even one. Along the way, however, assumptions are made, any of which could lead to a different conclusion.
Geologists estimate the Earth formed around 4.54 billion years ago. Yet, the origin of when and how life came to be on this planet is still unknown. Indeed, the earliest evidence of terrestrial life comes from fossils, known as stromatolites. These fossils are layers of single-celled microbes, known as cyanobacteria, discovered in Western Australia dating back to about 3.53 billion years. Since these bacteria were already complex organisms, the actual origin of life occurred much earlier. This is a “limit of horizon” problem and makes the scientific study of origins prior to single-cell microbes quite difficult (see Overcoming the Limits of Science). It also makes the natural chemical origin of life highly improbable.
As we explore the universe, its galaxies, stars, and exoplanets, we assume, given our limited understanding, that life requires unique circumstances to arise. One basic assumption is that the propitious location has to be within the habitable zone of a star. This means a location with a source of light, energy, liquid water, and biogenic elements such as carbon and other elements at the right temperature. The Earth certainly met those criteria.
From a basic thermodynamics perspective, life requires a constant source of power. Interestingly, life on Earth developed a single source of metabolic drive—that associated with transferring electrons by chemical reactions. Although living things can detect and generate other potential sources including magnetic, kinetic, gravitational, thermal gradient, and electrostatic, life used none of these for metabolic energy.
Second, carbon molecules gained the dominant role as the backbone of biochemistry for life. This is true not only on earth but, according to astrophysicists, the entire Solar System. Carbon is not only abundant, but the variety of chemical bonds it can form make it the basis of complex chains of different molecules. It is the LEGO brick of the chemical world. While carbon is necessary, it is not sufficient for life. An entire array of additional elements is needed for that, including water (H2O), nitrogen, sulfur, phosphorous, calcium, and potassium. There is no definitive list since it would depend on the organism considered (e.g., methanogens require high levels of nickel).
Third, any life based on molecules requires some kind of solvent to move around. Liquid is more ideal than gas or solid since water has unique physical and chemical properties making it well suited to support the complex chemistry required for life. For instance, water is the second most common molecule in the universe after hydrogen. Its ability to expand when it freezes keeps bodies of water from freezing into a solid. It can dissolve many substances easily and has a high heat capacity, which means it takes a lot of energy to change temperature. This is one major reason for the relatively moderate Earth climate.
Once these fundamental parts were in place, the next stage in the chemical origin of life likely involved the creation of complex molecules for a variety of functions. The most basic function being to convert energies into “food” and to organize, code, and sustain life. A variety of experiments have argued that life arose gradually from inorganic molecules, with “building blocks” called monomers, like amino acids and nucleotides, forming first. Monomers combined to polymerize or make more complex molecules called polymers, like proteins and nucleic acids. The last step required the creation of self-sustaining DNA and RNA polymers. This stepwise, spontaneous formation of simple, then more complex, then self-sustaining biological molecules—is still at the core of most origins-of-life hypotheses today.
Important questions remain. For instance, in living cells, enzymes put together polymers. Yet, enzymes are themselves polymers, so which came first is a kind of chicken-and-egg problem! One postulated solution is that monomers may have “spontaneously” formed polymers through some sort of catalytic accident. Experiments in the 1990s showed that RNA nucleotides link when exposed to clay, which acts as a template and catalyst to form an RNA polymer. However, another major roadblock is that polymerization is difficult in a watery environment. In fact, it requires the removal of water or dehydration. But, an even more troublesome issue is how polymers became functional and self-replicating. One possibility for replication is template-assisted ligation. However, one must also account for the ordering of amino acids in proteins and nucleotide bases in the RNA and DNA allowing them to function as sources of “information.” This ordering is what proponents of intelligent design call the “information sequence problem.”
Besides organizing, coding, and sustaining life, another major aim of these early complex molecules was to convert light into a food source or metabolic energy. Nature solved this problem with the appearance of light-activated enzymes. Light activated proteins are common, but light-activated enzymes are rare, with only three known, making this development one of the more tenuous points in the history of life’s origin. Protochlorophyllide oxidoreductase or ‘POR’, is the enzyme responsible for making the pigment vital for chlorophyll in plants. Without chlorophyll, there is no photosynthesis; without photosynthesis there is no plant life; and without plant life there is no us. Photosynthesis is the process that uses light energy to produce sugars from water and carbon dioxide, while emitting oxygen, which became so vital to human life.
Chlorophyll’s basic structure is a porphyrin ring with a magnesium atom at its center. This structure is, perhaps not unexpectedly, very similar to that of the heme molecule, which is found in the hemoglobin in our red blood cells, with an iron atom at its center, and which carries oxygen to every part of our bodies. Because of its structure, chlorophyll absorbs light in the blue and red parts of the visible spectrum, and reflects the green light back to our eyes. It is why plants appear green.
Hence, as we peer back in time to understand where we came from, our analyses lenses blur as events become indistinguishable, making scientific explanations of life origins extremely difficult. Even the distinguished materialist scientist, Francis Crick, who along with James Watson identified the structure of the DNA, said, “The origin of life appears to be almost a miracle, so many are the conditions which would have had to be satisfied to get it going.”
Perhaps because of such difficulties, another idea called “panspermia” has gained credibility. Organisms from one world get a ride to another to spread the organic seeds of life. Launched into space aboard discharged bits of planetary debris, these building blocks of life could, upon arrival at another planet, survive and thrive—perhaps evolving into the diversity of life we see on Earth. Recent analyses of Martian meteorites show several organic compounds including key building blocks of life, i.e., amino acids, nucleobases, and phosphate.
Or, then again, maybe life is a miracle!